Droplets atomization and transport in turbulent flows
From September 2016 to Juanuary 2020, in collaboration with
Alberto Aliseda.
Atomization of liquid jet arises in numerous industrial applications. Injection in gasifiers and spray coating for pharmaceutical tablets are some of the examples. Many of these applications are very sensitive to the droplet size. Therefore, there is a need to understand the underlying mechanism of the breakup of a liquid jet.
We are specifically interested in the gas-assisted atomization. Typically for this type of atomization, the liquid jet is surrounded by a high-speed annular gas stream. The breakup of the liquid jet is mainly determined by the interfacial interaction between the gas and the liquid phase. Our goal is to study this interaction and develop reduced-order models to implement real-time feedback control. This is done through detailed open-loop studies of the spray with different perturbations (swirl, electric or acoustic field...). Some of the experimental techniques we use for this study are high-speed flow visualization, Phase Doppler Particle Analysis and Synchrotron X-ray radiography. Videos
Inertial waves and turbulence in rotating fluids
From September 2014 to August 2016, in collaboration with
Pierre-Philippe Cortet and
Frédéric Moisy.
Motivation
A fluid rotating at constant angular velocity supports a specific and unusual class of waves, called inertial (or gyroscopic) waves, which propagate in the interior of the fluid. These waves are of primary importance in geophysical and astrophysical flows (in ocean, atmosphere and liquid core of planets, in rotating stars,...), in which cases they are often coupled with density stratification effects. Pure inertial waves are also relevant to industrial flows, such as spacecrafts fuel tanks or liquid-filled ballistics.
When the flow is turbulent, the effect of the Coriolis force is subtle: large scales may be dominated by the rotation, whereas small scales are not, because of their fast dynamics compared to the rotation rate. As a consequence, the large scales can be described as a system of superimposed inertial waves, leading to a partial two-dimensionalization of turbulence.
Inertial waves
Using the platform Gyroflow to rotate the fluid, we can excite inertial waves by applying an harmonic disturbance. The aim is to characterize the waves for different excitation modes, study the decay of theses waves and investigate the possible secondary mean flows.
Rotating Turbulence
In the same type of experiment as before, we can create turbulence by moving a grid for instance. We can then study fundamental aspects of turbulences such as the transition from three to two dimensionality, the inverse energy cascade and the anisotropic energy transfers.
Large particles in turbulent flow: transport, long-time dynamics and heat transfer
From September 2011 to August 2014, supervised by
Romain Volk and
Jean-François Pinton.
In collaboration with
Mickaël Bourgoin and
Javier Burguete.
Manuscript in
[pdf].
Motivation
A lot of real problems in fluid dynamics present turbulent conditions and the presence of particles. Those particles are often large, with density different from the fluid, having hence trajectories different from fluid particles. For example weather balloons transported by atmospheric current or solid particles dissolving in an industrial mixer, it is important to how the particles will behave and if they will present preferential sampling of the flow and/or long-time dynamics. We try to answer some of those questions with a Lagrangian study of large particles in a von Kármán flow.
Many of those problems also present mass or heat transfer, where, in turbulent conditions, the flow behaviour around the particles governs the transfer. After characterizing the dynamics of solid particles of the same density as ice, we study the melting of ice balls in a von Kármán flow.
Large particles in a turbulent von Kármán flow
To study the Lagrangian characteristics of large particles in a turbulent flow, we use a 2 cameras Particle Tracking Velocimetry setup. A fully developed turbulence is produced by two counter-rotating disks in a square section tank, giving Taylor micro-scale Reynolds numbers of the order of 500. The particles sizes are around 1000 times the Kolmogorov length scale, their diameters being of the order of the integral length scale of the flow.
With a high frame rate, we study the fast scale of the motion and obtain the particles velocity and acceleration statistics for different diameters, density ratio and Reynolds numbers. A large amount of data allows an Eulerian-Lagrangian conditioning, giving velocity or acceleration maps. We also track points on the particles surface to have access on the rotation of a particle on itself.
With a lower frame rate, we can only resolve the particles position, but the trajectories length can be of the order of 1000 times the integral time scale calculated with the impellers frequency. With this study, we can discuss the particles preferential sampling of the flow and their long-time dynamics. The different frame rates experiments can also be joined when looking at the particles positions power density spectra, which are resolved over 6 decades in time.
Melting dynamics of ice balls in a turbulent von Kármán flow
We study the melting dynamics of large ice balls in a turbulent von Kármán flow at very high Reynolds numbers with an optical shadowgraphy setup giving access to both particles tracking and size measurements. By recording the particle size variation along time, we compute the heat flux at the ice ball surface as a function of the particle scale Reynolds number.
Three cases of flow are studied: ice balls freely advected in the whole flow, ice balls fixed in a region of strong mean flow with lower fluctuations and ice balls fixed in a zero mean flow region with fluctuations of the same magnitude. We vary the ice balls initial diameter, the water temperature and the Reynolds number.
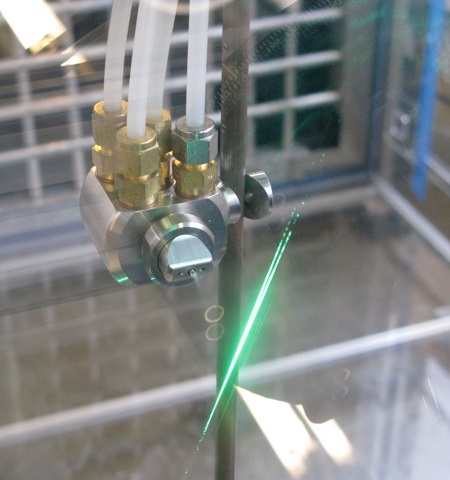
Spray droplets measured by a PPDA
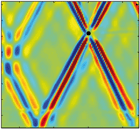
Inertial waves excitated by an oscillating cylinder
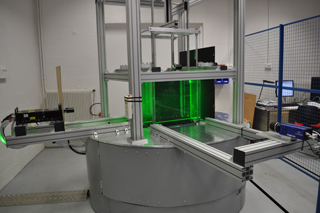
The Gyroflow platform
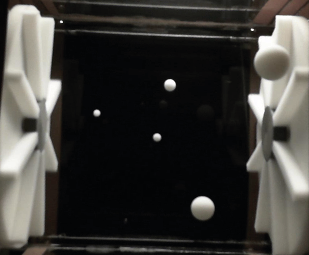
View of some particles in the von Kármán tank
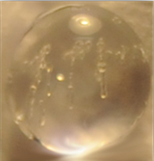
Picture of a home-made ice ball
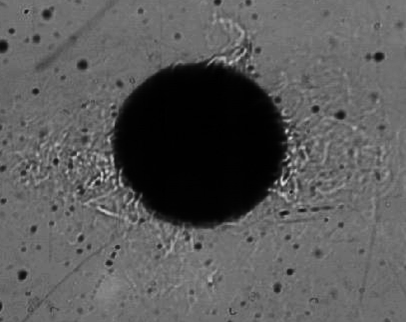
Shadowgraphic view of a melting ice ball